Biomaterials Processing
One applied goal of the materials we design is to mimic the structure and function of native tissues. A critical aspect for mimicking native tissues is recapitulating aspects of their complex spatial and temporal organization. In addition to our efforts to engineer the chemical details of biomaterials, we are also interested in processing methods that add complexity and hierarchy to their design. Traditional polymeric biomaterials often present design constraints that limit the geometric, surface, and interfacial properties of the materials. In our group, we utilize various techniques to fabricate materials with hierarchical structure. Toward this end, we utilize polymeric components as building blocks at different scales to process materials with spatial details at varying scales.
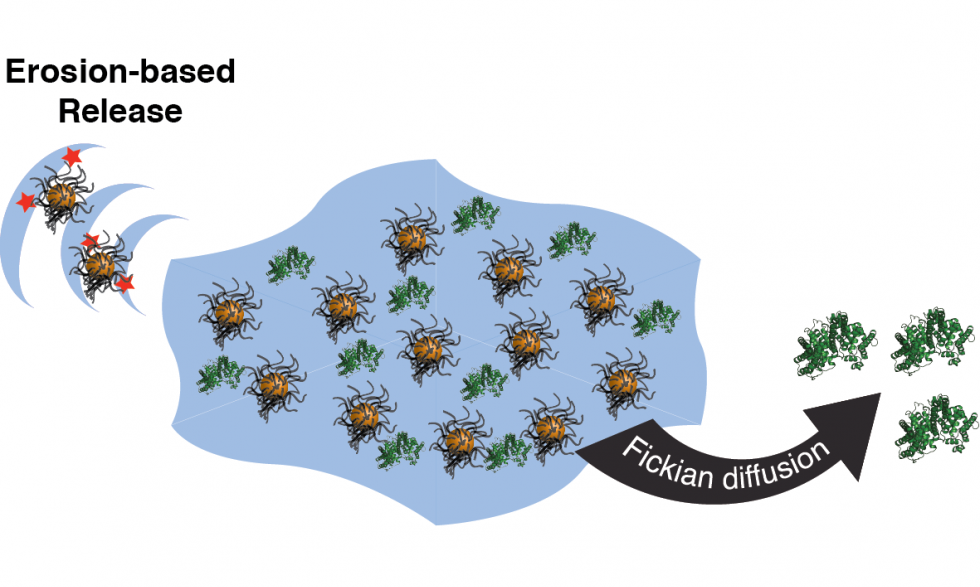
Nanoscale
At the nanoscale we produce and apply nanoparticles (NP) as material building blocks. Polymeric NPs can be used to assemble supramolecular hydrogels as well as for drug delivery. Methods for NP production that enable rapid formulation screening and continuous production are needed. Moving nanoparticle preparation from single batch towards a defined continuous manufacturing by flow controlled assembly allows precision and scalability.1 Encapsulation of cargo, such as therapeutic small molecules or biologics, for applications in drug delivery is enabled during this processing technique.
People involved: Elia Guzzi and Stéphane Bernhard.
Selected publications:
[1] Bovone et al., AIChE J. 2019, 65, e16840.
[2] Bovone et al., Front. Bioeng. Biotechnol. 2019, 7, 423.
[3] Tibbitt et al., J. Am. Chem. Soc. 2016, 138, 704.
[4] Fenton et al., Adv. Mater. 2016, 28, 2939.
Microscale
At the microscale we employ spatial patterning tools such as acoustofluidics (in collaboration with Prof. Dual’s group) to control spatial positioning of the cells within liquid hydrogel precursor for tissue engineering applications. Using stimulus-triggered polymerization, we can replicate cell organization in anisotropic tissues, like skeletal muscles or tendons. Moreover, we develop highly defined single cell microenvironments by photopatterning hydrogels with cell niches of distinct shapes and mechanical properties. Specifically, we have recently shown that biophysical properties of the cell niche and the extent of geometric confinement in 3D culture governs cell fate and function.1 We also use bottom-up approaches in hydrogel processing via the assembly of granular materials from micron-sized hydrogel building blocks. Fabrication of granular hydrogels enables us to control the porosity and stiffness of the materials independently, which when used as tissue mimics, can facilitate cell spreading, migration, and diffusion of nutrients.
People involved: Dhananjay Deshmukh, and Börte Emiroglu.
Selected publications:
[1] Dudaryeva et al., Adv. Funct. Mater. 2021, 2104098.
[2] LeValley et al., Coll. Surf., B 2019, 174, 483.
[3] Ragelle et al., Adv. Healthc. Mater. 2020, 9, 2001531.
Macroscale
At the macroscale we use extrusion based additive manufacturing (AM) as one of the ways to engineer the structure and mechanical properties of designed hydrogels. We can combine the hydrogel extrudability due to their shear-thinning and self-healing properties with different gelation mechanisms to fabricate organized 3D structures.1 Secondary gelation with light, pH, or temperature post-extrusion can be utilized to stabilize the scaffolds for their application in biomedical engineering like drug delivery devices or tissue mimics. Coupling several AM techniques to process a range of polymeric materials allows us to combine their properties and create composite structures.2
People involved: Dalia Dranseikiene and Yifan Cui.
Selected publications:
[1] Guzzi et al., Small 2019, 15, 1905421.
[2] Guzzi et al., Biofabrication 2021, 13, 044105.