Cell–material interactions
In every tissue, cells are embedded in a microenvironment composed of a fibrillar extracellular matrix (ECM), neighboring cells, and surrounding fluid. Cells dynamically sense the physical properties of the ECM (e.g., topographic features, stiffness), and mechanical forces (e.g., tensile, compressive, and shear forces). These physical signals are important for guiding cell proliferation and differentiation. We design tailored environments that can mimic aspects of these interactions to improve our understanding of cellular mechanotransduction and to foster translational applications in tissue engineering and regenerative medicine.
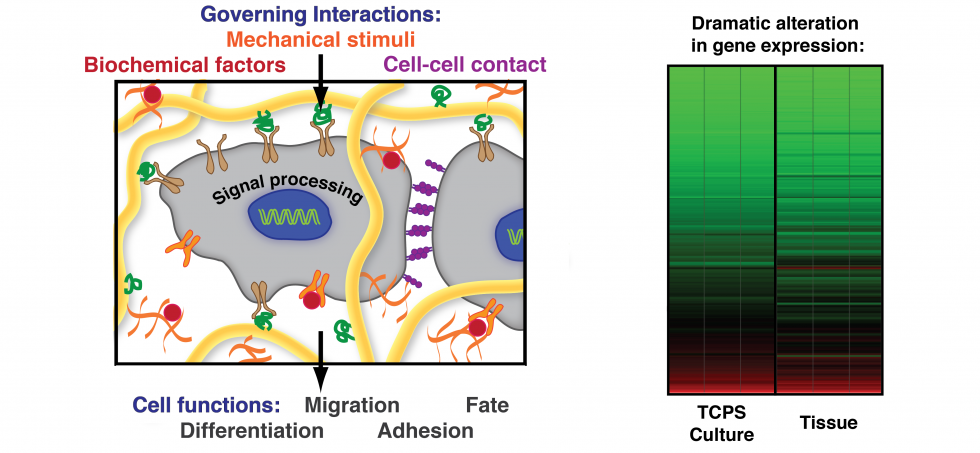
Tissue engineering
To closely mimic the 3D in vivo niche, we encapsulate cells in tailored hydrogels and use acoustofluidics to apply spatial patterning. In addition to designing the hydrogel biomaterial, we optimize the cell culture conditions and nutrient supply necessary for specific cells. Currently, we are working on optimizing tissue engineering platforms for musculoskeletal tissue1 and to induce the formation of vascular networks.
People involved: Dhananjay Deshmukh, Lorenza Garau Paganella.
Selected Publications:
[1] Deshmukh et al., Bioeng. & Transl. Med. 2020, 5, e10181.
[2] Guzzi et al., Biofabrication 2021, 13, 044105.
[3] Ragelle et al., Nat. Commun. 2018, 9, 1184.
Skin cancer models to replace xenografts
To study skin cancer and test potential therapies, researchers often use xenograft models, in which human skin cancer cells are transferred into mice, and the tumors are allowed to grow in the mice. This system relies heavily on animal experimentation. But alternatives exist, such as using hydrogels as 3D scaffolds for tumor spheroid growth. We are developing a biophysically and biochemically defined 3D in vitro models that would resemble a xenograft assay, allowing tumor growth and invasion. We are comparing different types of scaffolds, and will validate them by comparing the proteome of tumors in the different environments. The goal is then to use these assays to validate novel markers of tumor aggressivity.
This is a collaboration with the Dengjel group at University of Fribourg, and funded by the NRP79 program of the SNF.
People involved: Gabriela Da Silva André, Dr. Celine Labouesse.
3D models of the dermal niche
Skin is a 3D complex and hierarchical tissue, which plays a fundamental protective barrier role in the human body. We are developing 3D in vitro models to bridge the gap between animal studies and standard 2D cell culture. We use both synthetic hydrogels (e.g., poly(ethylene-glycol) based) and natural hydrogels (e.g., gelatin-based), of which we control the polymer weight and stiffness. We can trigger gelation after cell encapsulation using photosensitive initiators of cross-linking and/or enzymatic stiffening. These tools enable us to modulate the mechanical properties of the microenvironment and directly probe cellular response. We investigate how different physical (e.g. stiffness) and chemical (e.g. osmotic stress) stimuli affect the response of dermal fibroblasts. We combine expertise in hydrogel design with advanced biomechanics characterization and modelling to gain insight on the physical mechanisms underlying skin function and pathology.
People involved: Lorenza Garau Paganella and Dr. Céline Labouesse.
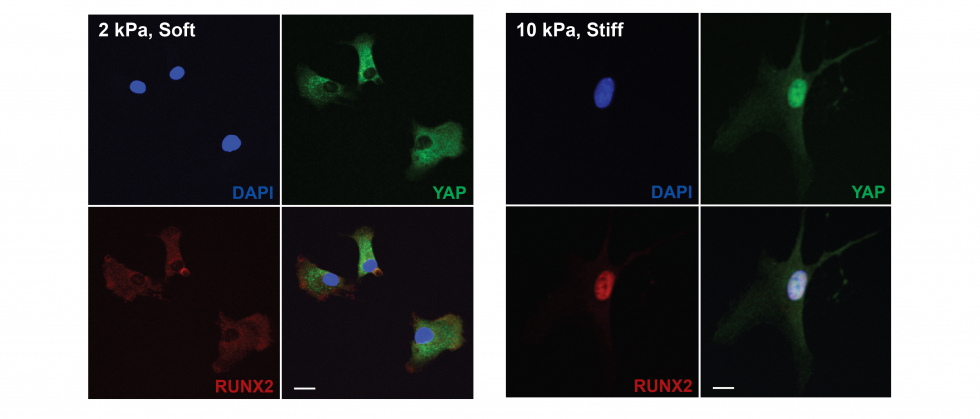
Cell mechanotransduction
Our collective knowledge of how cells sense their microenvironment through force-based interactions originates mostly from 2D studies. These have uncovered the mechanisms and pathways that control cell contractility, and mechano-regulation of gene expression, including the effect of mechanical memory1. In 3D contexts, cells develop lower forces and are under greater volumetric confinement.To understand the role of confinement in regulating cell function, we designed a microwell platform for 3D single cell niches of controlled size that can host single cells while restricting their spreading. We then measure how niche size and stiffness changes cell shape, cell adhesion, cell forces and downstream regulation of gene expression2. Cell-generated forces are estimated using Förster-based Energy Transfer (FRET) vinculin tension sensors. Ultimately, we will gain a better understanding of how mechano-transduction in 3D controls stem cell maintenance and differentiation.
People involved: Oksana Dudaryeva, Dhananjay Deshmukh, Céline Labouesse, Lorenza Garau Paganella
Selected Publications:
[1] Yang and Tibbitt et al., Nat. Mater. 2014, 13, 645.
[2] Dudaryeva et al., Adv. Funct. Mater. 2021, 2104098.